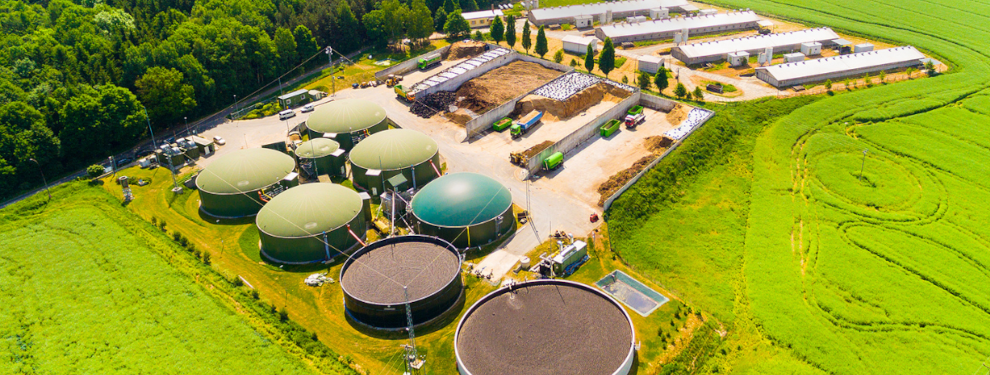
The history and technology of biogas: All About Biogas, Article #2
March 4, 2022 Biogas
In the first article in this series, we looked at the biogas industry and the regulatory environment in a number of Canada’s provinces. We will now look more closely at biogas technologies themselves, to better understand the promise and the limitations of biogas and its use in generating electricity, heat, and renewable natural gas (RNG).
A (very) Short History of Biogas
Biogas has been used on a small scale for heating, cooking, and lighting in low-tech environments around the world for centuries. Indeed, the World Biogas Association says biogas was used to heat Assyrian bath waters in 900 BC.1 However, the discovery of methane is generally attributed to an Italian scientist, Alessando Volta, in the 1770s – reportedly, his research was inspired by reading a paper by the American Benjamin Franklin on “flammable air”.2 Scientists found that methane was generated in swamps and rotting organic matter, a realization that led to the construction of the world’s first biogas digester in 1859 in Bombay, India. Soon after, sewer gases were being captured and used in Victorian England to provide street lighting.3
Modern biogas facilities have been in use since the 1930s, most notably in association with municipal sewage. Energy and waste interests led the technology’s growth through the 20th century, with biogas production most active in China, Germany, and the US.4 In the 21st century, biogas has grown faster, with supportive policies like Feed-In-Tariff programs (FIT) and clean fuel initiatives driving renewable energy in many jurisdictions. This has enabled an expansion of both small and large biogas digesters around the world.5
The collection and use of biogas, especially on a larger scale, has steadily improved with technological advances, including those in materials and design, computerization, automation and biological science. But these efforts to improve the capacities of modern biogas facilities add management complexity and additional costs. A modern biogas facility represents a substantial investment, and optimizing returns on that investment requires that each step in the process be carefully planned and managed.
How does a digester work? Biogas production is centred on anaerobic digesters, which may be located on a farm, an industrial site, or even attractions such as the Toronto Zoo. The digester is typically comprised of a round holding tank with a rubber bladder for a roof.
- Feedstocks are fed in steadily via a conveyor in a flow-through, or exchanged as a whole in a batch process.
- Gas is collected under the bladder, and fed as needed to a large gas-fired engine-generator set – or genset – usually located in a nearby building. Alternatively, the gas can be purified, odorized, and injected into the natural gas grid as biomethane, also called renewable natural gas (RNG).
- The genset produces electricity for the grid, and generates a fair bit of heat in the process.
- This heat is recycled to warm the digester, if needed, and excess heat is vented or, in a combined heat and power (CHP) arrangement, used to heat nearby water or indoor spaces. A schematic of a typical system is below.
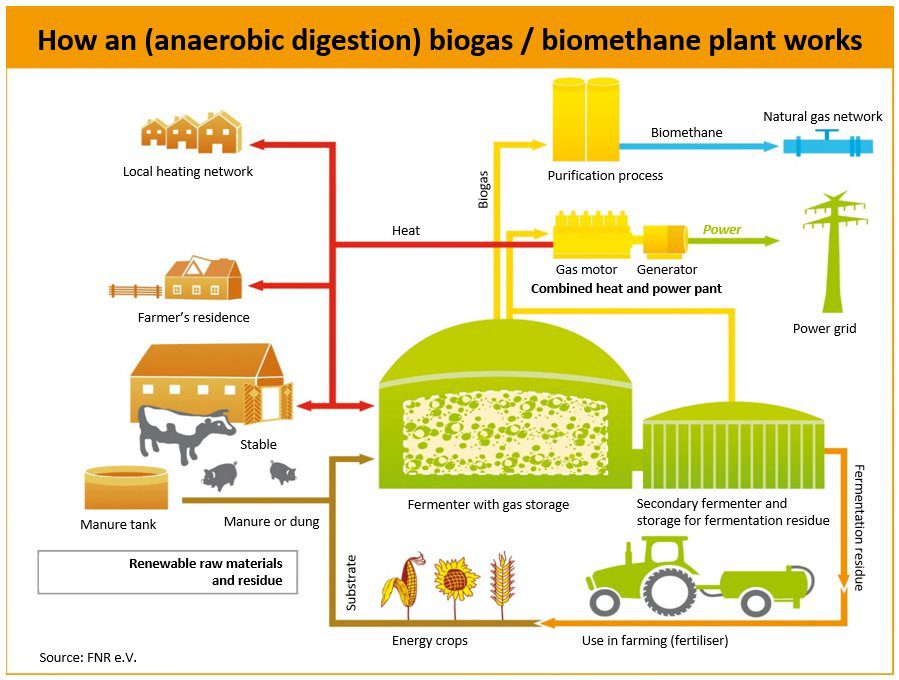
(Copyright: Creative Commons 4.0)
The nitty gritty on feedstocks
But before we dig into the digester, we need to think about the feedstock, or organic material, that goes into the process. Availability of suitable feedstock is not a trivial issue; a digester won’t run efficiently on just any old type of manure, and sufficient, reliable supplies of quality feedstock are not always readily available. Indeed, surveys conducted by the Canadian Biogas Association found that challenges around procuring quality feedstock are one of the principal barriers to the growth of biogas.6
Organic feedstocks vary widely in their digestibility, energy content, and availability. In an agricultural setting, manure is often a key component—not to mention a driving motivation–behind running a digester. Farmers value a digester for waste management, enabling them to process and dispose of animal wastes safely. They will often partner with local food processers to obtain higher energy inputs, which means these parties can dispose of their waste as well.
While primary crops like corn or beets provide some of the highest energy densities, stalks and greens generally have lower energy density; moreover, after an animal digests the organic matter, even less energy remains in its manure. Most on-farm biogas systems use a mix of animal and food wastes, including oils, while in Europe dedicated crops are commonly used.
Many on-farm digesters now accept source-sorted organic (SSO) municipal wastes – usually post-consumer organic/food wastes collected in “green bins” or bulk food waste (from grocery chains or processors) – while some take a wider range of feedstocks. For most digesters, it’s important to have a steady and fairly uniform feedstock supply, as the bacteria that break down the organic matter tend to be quite particular about maintaining their diet. Just as new foods can shock our own biotic system, you can’t simply switch a digester’s bacterial population from beets to banana peels without risking losses in efficiency and output.
Improving flexibility around biogas inputs is thus a key area of research, as companies work on second-generation biogas digesters that can deal with more irregular and mixed streams of materials. Bio-En Power, a leading developer in this space, has developed its own system – the one used in Skyline Clean Energy Fund’s recently acquired Woolwich Bio-En facility in Elmira, Ontario7 – that can turn municipal wastes with up to 25% inorganic contaminants into energy and a digestate acceptable for use on certified organic farms (http://www.bio-enpower.com/). As the company website says, such “second-generation digesters are the best choice for mixed commercial organic wastes, and for municipal ‘green bin’ collection programs, simply because these waste streams are so variable.” Increasingly, municipalities are looking to firms like Bio-En to process these waste streams, which indicates a promising area for market expansion.
Such growth is supported by the fact that biogas can be made using a very wide variety of materials ; this makes it more available than other substances like bio-ethanol (which requires high quantities of sugars, as found in corn) or biodiesel (which requires feedstocks with a high fat content). Biogas digesters tend to work better with the addition of such rich materials, but they can process a great many substrates – including the leftover wastes from bioethanol and biodiesel production.8
The Digestion Process
The conversion of biomass to methane proceeds through four different microbial processes: hydrolysis, acidogenesis, acetogenesis, and methanogenesis. These processes are the same regardless of the feedstocks used.
- Hydrolysis involves decomposition facilitated by the presence of water; as we know, wet stuff rots. The key here is that longer chain carbohydrates (fibres, sugars and other polymers) are broken down into shorter chains (monomers).
- In acidogenesis, these monomers are converted into fatty acids.
- Acetogenesis involves the uptake of these fatty acids and their conversion to acetic acid, along with carbon dioxide and hydrogen.
- Finally, in methanogenesis, the acetic acid and hydrogen are consumed, with the final products of the reaction being methane and carbon dioxide.
Along with the end products of methane and carbon dioxide, varying amounts of impurities are often found. These may include sulphuric compounds, heavy metals, or other toxins. Industrial and municipal wastes often contain appreciable levels of these, and they bring a number of risks, including the potential to disrupt or even destroy digester biota – resulting in a useless toxic sludge – or making the gas product unusable (such as for use in the gas grid). A trusted relationship between biogas operators and their feedstock suppliers is key to ensuring that all feedstock that enters the digester is of sufficient quality.
What are some differences between digester types?
Major factors that vary among digesters include:
- The water content of a biogas mixture can vary from a 60% “dry” mix to a 98% slurry. Low water content makes mixing and pumping of materials more difficult, so a wet mixture is most commonly used. But with a high water content, more heat must be added to maintain the digester’s temperature. Since this heat usually comes from the biogas produced (via the genset or burners), high water content means less fuel or heat is available as product.
- Digesters operate in the thermophilic (50-60 degrees Celsius), mesophilic (30-40 degrees Celsius), or, rarely, the psychrophilic (10-20 degrees Celsius) range. Thermophilic systems are generally more efficient, and most large digesters operate in this range, while most mid-sized systems run in the mesophilic range. The anaerobic reactions are largely endothermic, meaning they require heat to be added; moreover, heat losses can be substantial, especially in colder weather. The heat is usually provided from the genset, but in cold climates it can be a challenge to ensure systems maintain an appropriate operating temperature. Potential heat losses need to be carefully considered in plant design.
- Cycle times. These can vary from 1-2 days to a month, with warmer temperatures generally speeding up the process. Feedstocks can be fed in continuously or in batches. In second-generation plants these processes are usually separated, allowing for a wider range of feedstocks and, if done right, higher efficiencies.
- Efficiency. Like speed, efficiency tends to improve with heat and scale. But a study shows that the production of methane is also highly dependent on the types of bacteria present, and it can take a year or more to get a digester working optimally.9 The science surrounding these digestive bacteria is an area of research, as interesting questions remain around the digestion processes and how different bacteria strains perform.
The gensets themselves can vary in size and capacity, but all are effectively gas powered internal combustion engines in a stationary setting, usually housed in a dedicated building or a shipping container. The engines power a generator that converts the mechanical energy into electricity. Because biogas runs at lower efficiency per volume than natural gas (because of its CO2 content), manufacturers like Siemens and CAT have been moving toward dedicated lines engineered to accommodate varying methane/CO2 ratios. Current research is aimed at improving start-up times, durability, and handling varying gas mixtures, along with a wide range of controls.10 The corrosive effect of sulphuric compounds (from impurities in the biogas) is usually addressed through pre-treatment, but new solutions in oil technologies and venting can reduce the need and cost associated with removing these impurities.11
Stepping it up to Renewable Natural Gas
One of the most important advances in the biogas industry is the push to green the natural gas grid, an important step in reducing fossil-driven GHG emissions and offsetting consumption of fossil natural gas. A typical biogas methane concentration of 50-65% must be upgraded to 95% or better before it can be used in the gas grid or in natural gas vehicle engines. There are a number of techniques used to undertake the separation, including physical and chemical adsorption, differential pressure, and membrane separation (see figure). In each case the CO2 is separated out, leaving almost pure methane to pass through as renewable natural gas.
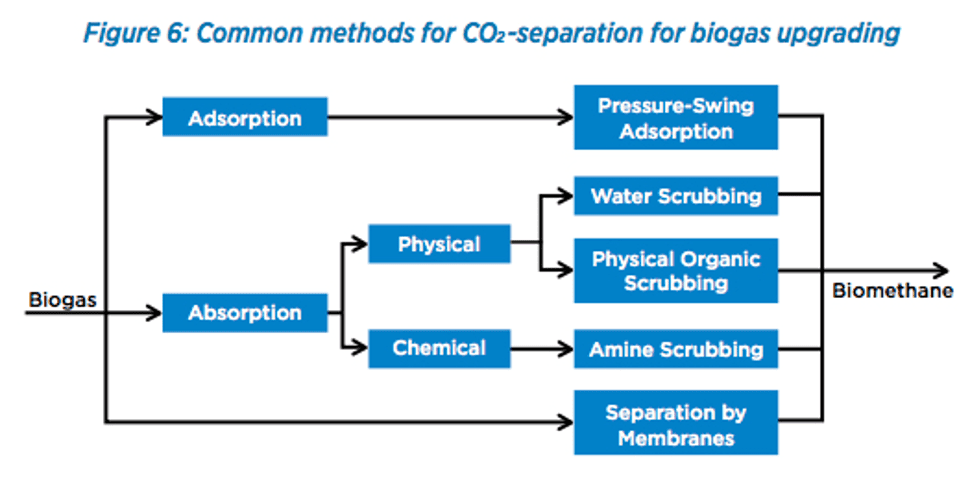
The purification process is simple enough, but the high capital cost of the necessary equipment means that this process is only viable within a larger facility. In addition, the purified gas – known as biomethane – needs to be treated and pressurized prior to being injected into the natural gas grid or transported. Depending on the proximity of the gas grid, this may involve kilometres of pipeline, adding further costs; bottling or trucking the gas is even more costly. For these reasons, smaller biogas facilities, and those far from a natural gas grid, are currently unlikely to contribute to RNG volumes. For larger facilities, proximity to the gas grid will remain an essential condition.
For many large food processors in southern Ontario, this wouldn’t be a big challenge. Industries that generate a lot of waste are already starting to invest in biogas digesters to reduce their waste and to generate electricity and process heat.13 Greenhouses, too, create a lot of uniform waste that can be digested to generate electricity and fertilizer, while also providing heat for off-season plantings. These facilities could potentially partner with companies that deal with restaurant grease or grocery chain waste, as farmers are already doing, to keep these wastes out of landfills.
The heat generated in the combustion process can be used for pre-heating in a wide range of industrial applications, or for space heating, allowing for almost endless opportunity for use. Architects, engineers, building managers and planners must all be involved in developments to maximize the opportunities for CHP systems, which have the potential to be integrated with district heating (as in the German “energy villages”), or used to provide power and heat in hospitals or community centres. While natural gas will usually be the cheapest and most available fuel, it makes sense to integrate biogas alongside or in place of natural gas where feasible.
Where biogas will further emerge in Canada
In the past few decades it has become increasingly clear to innovators that finding ways to convert waste into usable products is smart business, and anaerobic digestion of organics is poised to play an increasingly important part in a more energy efficient and ecologically sound economy. I believe new biogas technologies and deployment will continue to emerge in areas where policies support low carbon innovation and fossil fuel substitution, but also where grid connections, appropriate feedstocks, and creative thinking are present.
At the end of the day, there is only so much organic waste available, and location, transportation logistics, economics, and end product demand all play a role in the growth of the biogas sector. Yet the Canadian Biogas Association suggests that only 13% of Canada’s easily-tapped biogas potential is currently being used14; a 2020 Natural Resources Canada (NRCan) study found that the total possible output of biogas could provide the same energy as 100 large dams.15 Tapping into that energy could mean big changes to Canada’s energy landscape.
The information contained within is disseminated by Skyline Wealth Management Inc. (“Skyline Wealth Management”) on behalf of the Issuer as at the date of publication and Skyline Wealth Management does not undertake to advise the reader of any changes. The opinions and statements expressed within are of those of the Issuer and do not necessarily reflect those of Skyline Wealth Management. Skyline Wealth Management has not taken any steps to verify the accuracy or completeness of the information provided herein.
Skyline Wealth Management Inc. (“Skyline Wealth Management”) is an Exempt Market Dealer registered in the provinces of Alberta, British Columbia, Manitoba, New Brunswick, Newfoundland and Labrador, Nova Scotia, Ontario, Prince Edward Island, Quebec and Saskatchewan. The information provided herein is for general information purposes only and does not constitute an offer of securities. Sales of interests in any investments offered by Skyline Wealth Management are only made to certain eligible investors pursuant to regulatory requirements and available exemptions. Any information provided herein is current as at the date of publication and Skyline Wealth Management does not undertake to advise the reader of any changes.
References
- Why biogas? World Biogas Association. (2020, May 6). Retrieved March 3, 2022, from https://www.worldbiogasassociation.org/why-biogas/
- Wikipedia contributors. (2001, September 16). Alessandro Volta. Wikipedia
- Short History of Anaerobic Digestion.” Penn State Extension, 8 Feb. 2022, https://extension.psu.edu/a-short-history-of-anaerobic-digestion.
- IRENA (2018), Biogas for road vehicles: Technology brief, International Renewable Energy Agency, Abu Dhabi. https://www.irena.org/publications/2017/Mar/Biogas-for-road-vehicles-Technology-brief
- A global perspective of anaerobic digestion policies and … (n.d.). Retrieved March 4, 2022, from https://development.globalmethane.org/documents/tools/A-Global-Perspective-of-AD-Policies-Incentives.pdf
- The full report is available to members of the Association, and the summary report can be requested. The key data are also summarized at https://mcusercontent.com/881b9099f1cf2d8e98772c098/files/713e79d0-07a3-89a5-cce2-1d96d623f53d/Canadian_2020_Biogas_Market_Full_Report.pdf
- Skyline Clean Energy Fund has an 80% ownership stake in SBE LP, and SBE LP holds a biogas facility that has 110,000 tonne organic waste processing capacity.
- Biogas – Feedstocks. (2019). Biogas a Renewable Biofuel. https://biogas.ifas.ufl.edu/feedstocks.asp
- Zhang et al., 2019. “High variations of methanogenic microorganisms drive full-scale anaerobic digestion process.” Environment International 126, May, pp. 543-551, https://pubmed.ncbi.nlm.nih.gov/30852441/; also Kerstine Appunn, 2016, “Bioenergy – the troubled pillar of the Energiewende”, Clean Energy Wire, 30 September. https://www.cleanenergywire.org/dossiers/bioenergy-germany
- See, for example, https://assets.siemens-energy.com/siemens/assets/api/uuid:f85d6e08-02c8-4a0a-a881-2d274369cdb7/p_ldora-4—new-developments—r-d—subttulos-espaol.mp4 ; https://www.cat.com/en_US/by-industry/electric-power/electric-power-industries/agriculture.html
- https://assets.siemens-energy.com/siemens/assets/api/uuid:f85d6e08-02c8-4a0a-a881-2d274369cdb7/p_ldora-4—new-developments—r-d—subttulos-espaol.mp4 ; https://www.cat.com/en_US/by-industry/electric-power/electric-power-industries/agriculture.html
- IRENA (2018), Biogas for road vehicles: Technology brief. International Renewable Energy Agency, Abu Dhabi, p. 19. (Free to use with attribution.)
- Stormfisher announces $20M facility in Southwestern Ontario. Bio Market Insights. (2021, July 28). Retrieved March 4, 2022, from https://biomarketinsights.com/stormfisher-announces-20m-facility-in-southwestern-ontario/
- The full report is available to members of the Association, and the summary report can be requested. The key data are also summarized at https://mcusercontent.com/881b9099f1cf2d8e98772c098/files/713e79d0-07a3-89a5-cce2-1d96d623f53d/Canadian_2020_Biogas_Market_Full_Report.pdf
- Canadian Biogas Association, 2020 Canadian Biogas Market Report, Summary, p. 9.
About Skyline Clean Energy Fund
Skyline Clean Energy Fund (“SCEF”) is a privately owned and managed portfolio of medium to large scale clean energy assets, focused on acquiring income-producing assets across Canada, under long-term government contracts.
SCEF is offered as an alternative investment product through Skyline Wealth Management Inc. (“Skyline Wealth Management”), the exclusive Exempt Market Dealer (EMD) for SCEF.
SCEF is committed to surfacing value to its investors through asset monitoring and optimization, while providing a sustainable investment product based in clean, renewable energy.
To learn more about SCEF and its asset manager, Skyline Energy, please visit SkylineEnergy.ca.
To learn about additional alternative investment products offered through Skyline Wealth Management, please visit SkylineWealth.ca.
Skyline Clean Energy Fund is operated and managed by Skyline Group of Companies.
For media inquiries, please contact:
Cindy BeverlyVice President, Marketing & Communications
Skyline Group of Companies
5 Douglas Street, Suite 301
Guelph, Ontario N1H 2S8
cbeverly@skylinegrp.ca
More from this category:
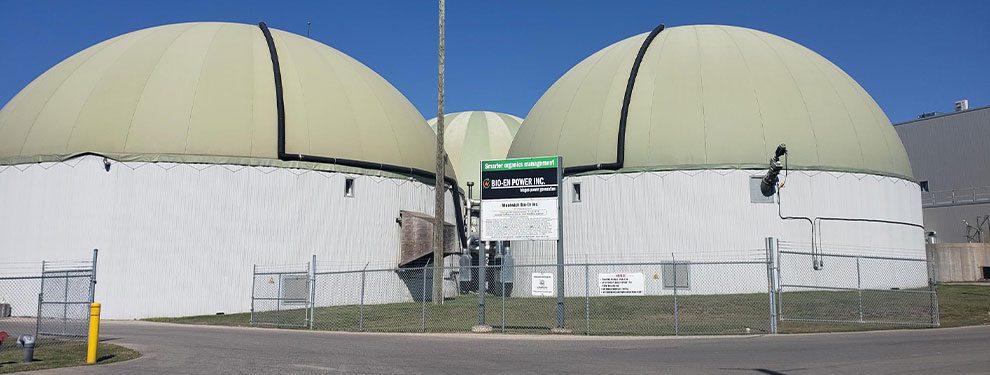
Skyline Clean Energy Fund Purchases Biogas Facility
[Guelph, Ontario – August 24, 2021] On August 24, 2021, Skyline Clean Energy Fund (the “Fund”) purchased its first biogas clean energy asset. The 2.85 MW/DC1 biogas facility, located in Elmira, Ontario, was originally developed and built by Woolwich Bio-En Inc. (WBEI). Skyline Clean Energy Fund purchased an 80% stake in the facility for $43.2M. [...]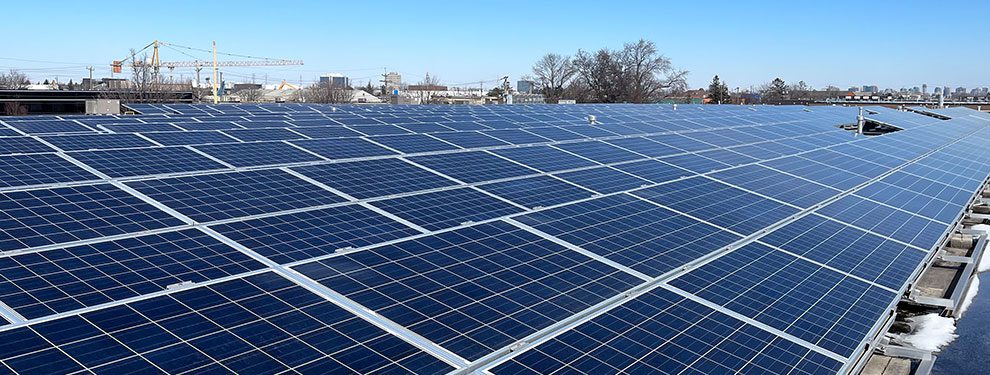
Canadian Clean Energy Fund Looks Back on Record Year; Anticipates Growing Energy Demand in 2023
[Guelph, Ontario – January 13, 2023] Skyline Clean Energy Fund (SCEF), a Guelph, Ontario-based equity growth fund investing in clean energy assets, is reporting several landmark achievements in 2022 and is looking to capitalize on further growth opportunities in the new year. In 2022, SCEF closed six transactions, increasing its Expected Annual Generation to 125,327 […]
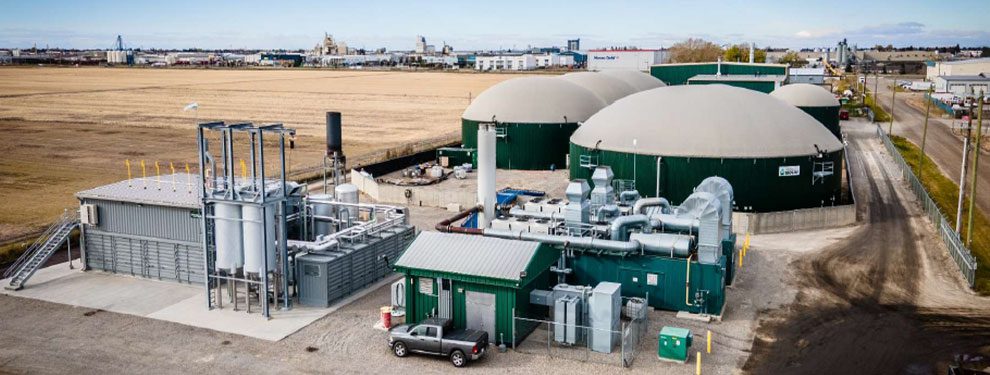